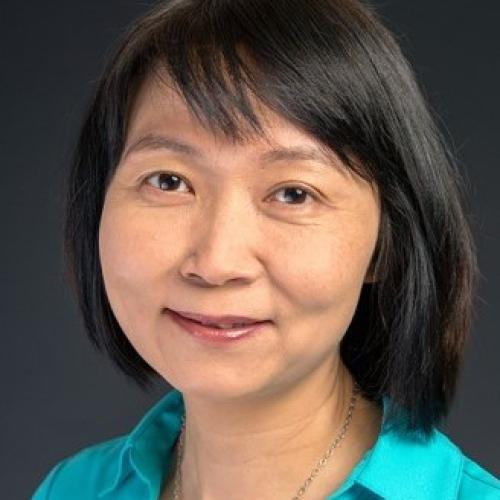
Professor, Physics, UT Austin
IRG 2 Co-Leader
Faculty Investigator, IRG 2
I knew that I wanted to teach after graduating from high school. I went to Beijing Normal University thinking that I would become a high school physics teacher. While in college, one of my professors strongly encouraged me to go to the U.S. for graduate studies. I was young and very interested to see a different part of the world. I thought “what the heck, it might be fun.” So I went to the University of Michigan, where I visited some experimental research groups. My impression of the first lab I ever visited was “Boy, this place is messy!” I still decided to study experimental physics because I did not like the idea of sitting in a chair all day long. My choice of a particular lab was also based on a couple of somewhat random factors: First, I like the idea of working with lasers since I was always fascinated with laser shows. Second, I thought my advisor seemed like a kind person. He reminded me of “Santa Claus.” I was frustrated most of the time in graduate school. I now understand that all those struggles were just typical “growing pains” for a young researcher.
After spending six years at Michigan, I went to JILA in Colorado (a leading research institute for optics-related fields) as a postdoctoral fellow. I thoroughly enjoyed my years at JILA. Although I also struggled with various research projects, I was used to wrestling with scientific obstacles by then. I worked very hard during the week and spent the weekends hiking and skiing. I joined UT Austin in January 2007. I often think that I have the perfect job. I enjoy both teaching and research. It takes a lifetime of continuous effort to become both a good teacher and a good researcher. There will never be a dull moment.
What I like about physics is its universal applicability and elegance. Simple conservation laws hold at both the smallest sub-atomic scale and the largest astronomical scale. What I enjoy about research on a daily basis is that you are always solving puzzles in the lab. (Yes, I like playing Sudoku.) Although certain types of jobs in the lab are repetitive and a bit boring, the idea that my work will eventually lead to the discovery of new knowledge always excites and motivates me.
My research focuses on studies of the quantum dynamics of electrons. To meet the technical and societal challenges of the new millennium, scientists need to learn how to control material properties at the level of electrons. To see the relevance of our research, one needs to look no further than the transistor. The transistor is arguably the most important invention of the 20th century. Transistors are essentially switches, which have an “on” and an “off” state. These two states can conveniently represent binary information, which consists of only “0” and “1.” The transistor is an essential component in all electronics, including the computer you are using to access this information. The lateral size of a transistor in the first commercial microprocessor, Intel’s 4004 (released in 1971), was only about 10 microns. As a reference, the diameter of a human hair is about 100 microns. The size of a transistor has kept shrinking ever since, from 1 micron in 1985, to 0.1 micron in 2002, to tens of nanometers in current electronics. This miniaturization has enabled faster and more compact devices. If this trend continues, transistors will reach the size of individual atoms in a decade or two. What happens then? The quantum dynamics of electrons will rule! Understanding how to probe and even control electron dynamics in individual quantum systems, therefore, might hold the key to future technological challenges.
The tools used by our group to investigate electron dynamics are lasers. There are several reasons for this. First, photons are one of the most non-invasive probes. Second, we use ultrafast laser pulses, which last only ~10-13 of a second, to capture extremely fast electron dynamics in materials. Imagine that you are trying to capture a quickly moving object with a camera. You need a fast shutter. Otherwise, the image will be blurred. Ultrafast laser pulses are the shortest events ever created and measured. Finally, the phase coherent nature of lasers allows us not only to probe but also to control electron dynamics, which is a holy grail for light–matter interaction studies.